Introduction to Protein Types
Proteins occur in two primary forms: globular and fibrous. These protein structures differ in shape and their unique roles within the body, making them indispensable.
Globular proteins are generally compact, spherical, and soluble in water. They perform various functions thanks to their flexible structures. Examples include hemoglobin, the oxygen-carrying molecule found in red blood cells, and enzymes like amylase, which catalyze biochemical reactions essential for processes like digestion.
Fibrous proteins are elongated, rope-like, and typically insoluble in water. They provide structural support and strength to cells and tissues. Keratin, found in hair, nails, and the skin's outer layer, offers these tissues rigidity and strength. Collagen, a critical component of connective tissues like tendons and ligaments, offers tensile strength and elasticity.
Fibrous Proteins
Keratin is a robust fibrous protein found predominantly in hair, nails, and the outer layer of the skin, providing these tissues with rigidity and protection. Its tightly packed filaments form a highly durable and inert structure resistant to wear and tear.
Collagen, the most abundant protein in the human body, is primarily found in connective tissues such as tendons, ligaments, skin, and cartilage. It provides tensile strength and maintains the structural integrity and elasticity of connective tissues. Collagen molecules are organized into a triple-helix structure, further cross-linked into fibrils and fibers that are incredibly strong yet flexible.
Elastic fibers, composed of elastin and other proteins, contribute significantly to the elasticity and resilience of tissues like the lungs, arteries, and certain ligaments. Elastin's unique properties allow tissues to stretch and return to their original shape.
Fibrous proteins are crucial in tissue repair and regeneration, such as fibroblasts upregulating collagen synthesis in response to tissue injury, facilitating wound healing. Disruption in collagen production or assembly can lead to conditions like osteogenesis imperfecta, where bones are extremely brittle due to defective collagen.
The stability of fibrous proteins is largely due to various chemical bonds and interactions, including disulfide bridges, hydrogen bonds, and hydrophobic interactions, ensuring they maintain their structural integrity under physiological conditions.
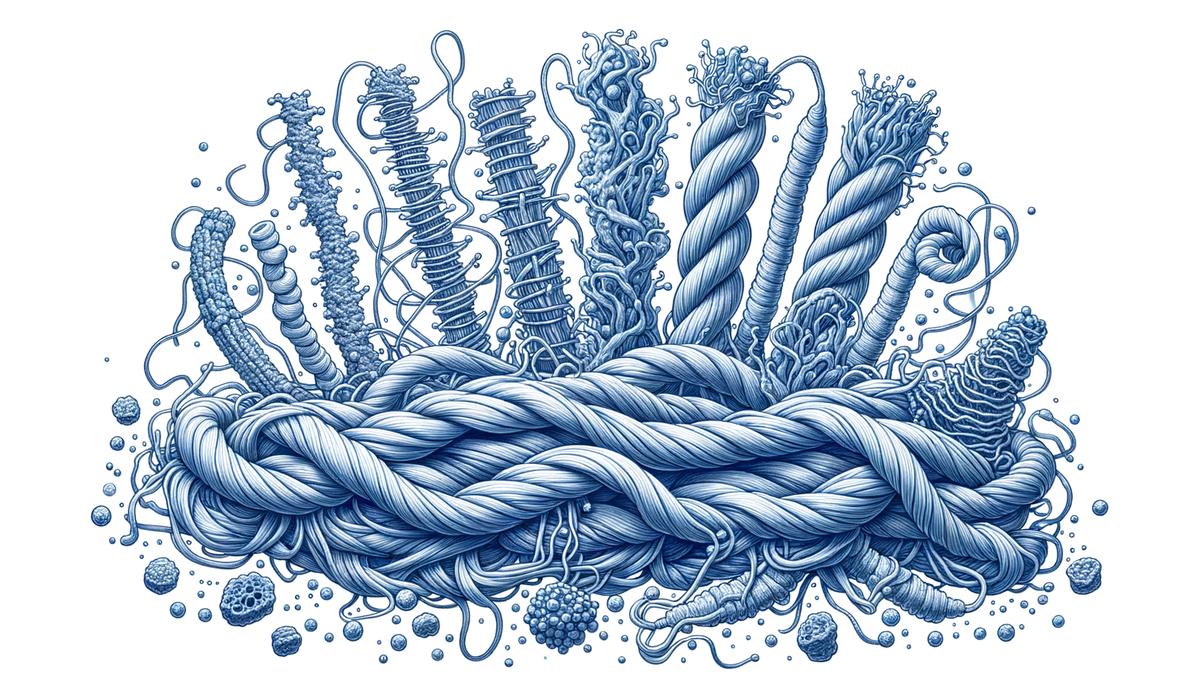
Globular Proteins
Globular proteins are soluble in water, allowing them to readily participate in various biochemical processes. Their three-dimensional folding positions hydrophilic residues on the exterior, interacting favorably with the aqueous environment, while hydrophobic residues are tucked inside.
Enzymes, like salivary amylase, are globular proteins that catalyze biochemical reactions. Each enzyme is highly specific, acting on a particular substrate and facilitating a specific type of chemical reaction. This specificity is due to the unique three-dimensional structure of the enzyme, which includes an active site where substrate binding and reaction occur.
Hormones, such as insulin, are crucial globular proteins that regulate physiological processes. Insulin promotes the uptake of glucose by cells, maintaining blood sugar levels within a healthy range. Imbalances in insulin levels, as observed in diabetes, can have severe metabolic consequences.
Transport proteins like hemoglobin and albumin play pivotal roles in transport processes. Hemoglobin transports oxygen from the lungs to tissues, while albumin carries various substances, including hormones, vitamins, and drugs, and helps maintain the osmotic pressure of the blood.
The folding of globular proteins, driven by chemical interactions like hydrogen bonds, ionic interactions, and hydrophobic interactions, is key to their functionality. Misfolding can lead to loss of function and is associated with diseases like Alzheimer's, where misfolded proteins accumulate in the brain.
Protein Classification by Function
- Enzymes are biological catalysts that accelerate chemical reactions within the body, facilitating essential biochemical processes like digestion and DNA replication.
- Transport proteins facilitate the movement of molecules across cell membranes and within the bloodstream, such as hemoglobin transporting oxygen and transferrin carrying iron.
- Structural proteins provide support and shape to cells and tissues, including collagen, keratin, actin, and tubulin.
- Hormones are signaling proteins that regulate physiological processes, like insulin regulating glucose metabolism and thyroxine regulating metabolism and growth.
- Defense proteins protect the body from pathogens, such as immunoglobulins (antibodies) identifying and neutralizing foreign invaders, and complement proteins enhancing the immune response.
- Contractile proteins, like actin and myosin, are involved in movement and muscle contraction, converting chemical energy into mechanical work.
- Storage proteins serve as reservoirs for essential nutrients and ions, including ferritin storing iron, casein providing amino acids and minerals, and ovalbumin providing nutrients for embryonic development.
Protein Structure and Function Relationship
Proteins showcase a remarkable connection between their structure and function. The four levels of protein structure—primary, secondary, tertiary, and quaternary—dictate their specific roles and biological functions, with each level building upon the previous, resulting in complex molecules capable of diverse essential life tasks.
The primary structure, the unique amino acid sequence, governs the arrangements and functions. A single alteration, like substituting one amino acid, can lead to significant functional changes. In sickle cell anemia, replacing valine for glutamic acid in hemoglobin's beta chain causes abnormally shaped red blood cells that can obstruct vessels, leading to health issues.
Secondary structures like alpha helices and beta sheets, stabilized by hydrogen bonds, contribute to proteins' properties. The alpha helix's coiled shape provides rigidity and tensile strength to keratin, found in hair and nails. Beta sheets, with strands connected by hydrogen bonds, are common in fibrous silk fibroin, contributing to elasticity and strength.
The tertiary structure, the polypeptide chain's three-dimensional folding, is crucial for specific functions. Enzymes like chymotrypsin have a particular tertiary structure forming active sites where substrates bind and undergo reactions. Disruption like denaturation can result in loss of function, highlighting the importance of precise folding for enzymatic activity.
The quaternary structure involves assembling multiple polypeptide chains or subunits into a functional complex, stabilized by interactions similar to tertiary structures. Hemoglobin, with four subunits working together to transport oxygen, exemplifies this. Changes in subunit interactions can affect oxygen-binding capacity, demonstrating quaternary structures' collaborative nature in functionality.
Collagen's unique triple-helical quaternary structure provides exceptional tensile strength for connective tissues like tendons and skin. The tight fibril packing and cross-linking enable tissues to withstand stretching forces, underlying collagen's critical mechanical properties.
Globular proteins' intricate folding allows diverse functions. Insulin's specific tertiary structure enables tight cell surface insulin receptor binding, triggering glucose uptake, ensuring the necessary interaction for its regulatory metabolic role.
Antibodies illustrate structure's significance in immune defense, with a quaternary structure of two heavy and two light chains forming antigen-binding sites. The variable regions produce a vast antibody array, each binding a unique antigen, allowing the immune system to recognize and neutralize pathogens.
Understanding these relationships underscores the elegance of biochemical processes at the molecular level, highlighting protein structure's profound impact on life's myriad functions.
Mutations and Protein Function
Mutations in proteins' amino acid sequences can profoundly impact their function, often leading to diseases and disorders. These changes typically result from genetic mutations due to DNA replication errors, exposure to mutagens, or inherited genetic variations. The impact on protein function can range from minor activity alterations to complete loss of function, subsequently disrupting cellular and physiological processes.
- Sickle cell anemia results from a single nucleotide mutation in the hemoglobin gene, causing abnormal hemoglobin S production, which polymerizes under low oxygen conditions, deforming red blood cells into a sickle shape, obstructing blood flow.
- Cystic fibrosis results from mutations in the CFTR gene, which codes for a chloride channel protein. The ΔF508 mutation causes the CFTR protein to misfold, preventing it from reaching the cell membrane, disrupting ion balance and causing thick mucus buildup in the lungs and other organs.
- In phenylketonuria (PKU), mutations in the PAH gene encoding the phenylalanine hydroxylase enzyme lead to reduced or absent enzyme activity, causing phenylalanine accumulation in the blood, which is toxic to the brain and can cause intellectual disability.
- In certain cancers, mutations in proto-oncogenes or tumor suppressor genes result in malfunctioning proteins that drive uncontrolled cell proliferation. Mutations in the TP53 gene, encoding the tumor suppressor protein p53, prevent it from carrying out DNA repair or inducing cell death, allowing cells with damaged DNA to continue dividing, leading to tumor development.
- Alzheimer's disease involves the accumulation of misfolded amyloid-β peptides and hyperphosphorylated tau proteins. Mutations in the amyloid precursor protein (APP) gene or in presenilin genes can lead to overproduction or improper processing of amyloid-β, promoting plaque formation that disrupts neuronal function and contributes to cognitive decline.
Studying protein mutations is crucial in understanding the molecular basis of diseases and developing targeted therapies like imatinib, designed to inhibit specific mutant proteins' activity, such as the BCR-ABL fusion protein in chronic myeloid leukemia (CML).
Overall, mutations can lead to loss of function, gain of abnormal function, or dominant-negative effects, where a mutant protein interferes with the wild-type protein's normal function. Understanding these mechanisms helps identify disease biomarkers and design precision medicines that specifically target dysfunctional proteins, providing more effective and personalized treatment options.
Understanding the intricate relationship between protein structure and function reveals how these macromolecules perform a vast array of essential tasks, acting as catalysts, transporters, defenders, and structural supporters—all integral to maintaining life's complex machinery.
Leave a Reply